VSEPR Supporting Evidence
The test of a good model, is how well it stands up to
experimental data. How well does VSEPR work? We can evaluate
several ways. One way we will use is related the polarity of
compounds. That is, how well does VSEPR predict the polarity of
molecules and how do experimental observations compare. What do I
mean by polarity of molecules? A molecule is polar if as a result
of unequal sharing of electrons, centers of positive and negative
charge do not coincide. Recall in CHEM 1215 we discussed the
polarity of chemical bonds in simple binary compounds. The
criteria for determining whether a chemical bond is polar or
nonpolar was the difference in the electronegativity of atoms
involved in the chemical bond. If the two atoms differed in
electronegativity the chemical bond was polar. If the
electronegativity was the same the bond is nonpolar.
We conclude that F2 has a nonpolar bond
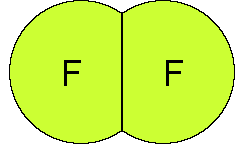
because the electronegativity is the same for the two atoms
sharing the pair of electrons in the bond. For HF the two atoms
are different,
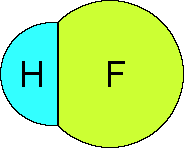
Fluorine is more electronegative compared to
hydrogen. The result is the electrons in the covalent bond spend
more time on the fluorine atom than on the hydrogen atom. This
produces a partial negative charge on the fluorine and a partial
positive charge on the hydrogen.
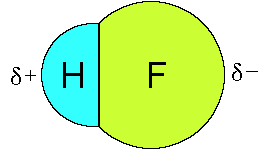
This separation of charge produces a small
dipole making the molecular polar. The molecule can be thought of
as a small magnet with a positive end and a negative end.
Opposites attract so HF molecules are attracted to each other
when in the liquid and solid phase (but more about that later).
The dipole that is produced is represented as a vector with an
arrow pointing towards the negatively charged end of the molecule
and a positive symbol on the other end of the vector. (The
magnitude of an electric dipole is reported as the electric
dipole moment, in a unit called the debye (D).)
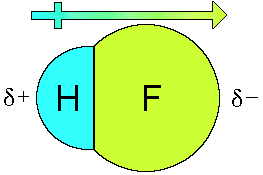
So we conclude that HF is a polar molecule because the only
bond in HF is polar. F2 is a nonpolar molecule because
it does not contain a polar bond. All heteronuclear diatomic
molecules are polar and all homonuclear diatomic molecules are
nonpolar.
The presence or absence of an electric dipole can be
determined experimentally if a sample of the compound of interest
is placed in an electric field. If the molecule is polar
alignment with an electric field will occur. The net result is
the molecules effect the capacitance (the capacity of the
plates to hold a charge) of the plates which maintain the
electric field. By measuring the capacitance of the plates
separated by different chemical substances allows the
determination of dipole moments. If a molecule can be
characterized as having polar bonds that do not cancel each other
out the compound will have an experimentally measureable dipole
moment.
What happens in more complicated molecules? It turns out
that, depending on the geometry, these individual polar bonds in
molecule may cancel each other out, which results in a nonpolar
compounds. If the polar bonds in a molecule do not cancel each
other the molecule is polar and contains a permanent distribution
of electron density which gives rise to a dipole moment.
Lets consider several examples to demonstrate how the
geometry of a molecule effects the dipole moment of a compound.
We'll begin with CO2 and H2O. Both molecule
have a central atom, carbon or oxygen, surrounded by two terminal
atoms. According to VSEPR CO2 is linear and H2O
is bent. If we consider CO2 each C=O bond is polar,
because of the difference in electronegativity of the atoms in
the bond. However, because the geometry of the two polar bonds
are exactly opposite one another and equal in magnitude the net
effect is to cancel each other out so that the molecule has no
dipole moment, CO2 is a nonpolar compound.
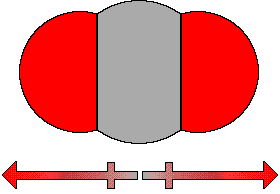
H2O, on the other hand, which also contains two
polar bonds (O-H) has a molecular geometry that does not allow
the contributions of the polar bonds to cancel out. Water has a
permanent dipole moment and is a polar compound.
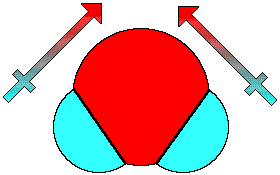
Experimentally CO2 has no measureable dipole
moment, but H2O (1.87 x1018 D) has a
very large dipole moment. We will discuss how the polarity of
water effects its physical properties in Chapter 14.
We can generalize these two cases.
Molecules containing central atoms with no lone pair
electrons and bonding pairs of electrons to identical
terminal atoms are nonpolar,
Molecules containing central atoms with one or more
lone pair electrons are polar. (Note: there are
exceptions to this rule for central atoms with
electron-pair geometries of trigonal bipyramidal and
octahedral. But we will not discuss these exceptions.)
Lets consider two additional examples to demonstrate how the
geometry of a molecule effects the dipole moment of a compound.
We will consider CCl4
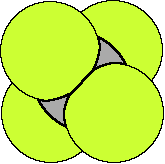
and CH3Cl.
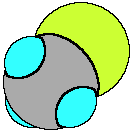
Both molecule have a central carbon atom, surrounded by four
terminal atoms. According to VSEPR both CCl4 and CH3Cl
are tetrahedral. If we consider CCl4 each C-Cl bond is
polar, because of the difference in electronegativity of the
atoms in the bond. However, because the geometry of the four
polar bonds and that all four bonds are equally polar the net
effect is to cancel each other out so that the molecule has no
dipole moment, CCl4 is a nonpolar compound. CH3Cl,
on the other hand, which also contains polar bonds (C-H and C-Cl)
is also tetrahedral, however because the terminal atoms are non
identical the compound has a permanent dipole moment and is a
polar compound. This introduces one more rule for predicting
polar compounds,
Anytime the molecule has a central atom with non
identical terminal atoms the molecule will be polar.
After all this if I give you the formula of a compound you
should be able to
draw the Lewis structure;
then tell me
the number of lone pair and bonding
pair of electrons on the 'central' atom;
the electron-pair and the molecular
geometry;
the bond angles around any 'central'
atom; and
if the molecule is polar or nonpolar.
So lets try figuring out the polarity for BF3 and
NH3. Go here to see what I
came up with!